Self-calibrating quantum sensor a game changer for vacuum measurement
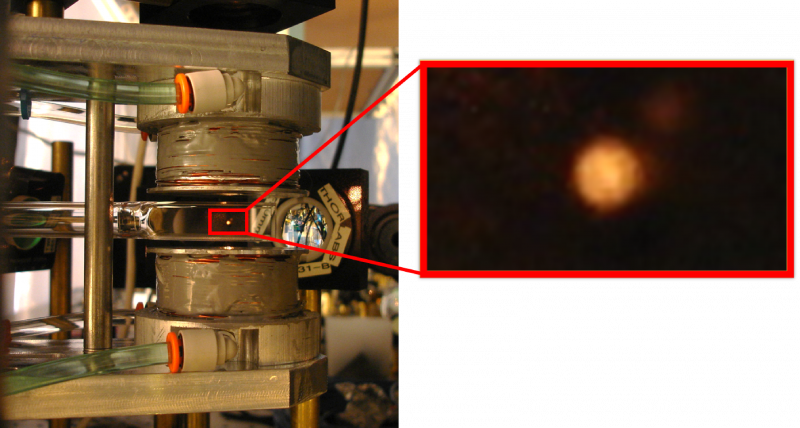
Dreams of harnessing quantum mechanics to realize secure communications, exponentially powerful computational machines, novel materials, and enhanced sensing modalities have inspired entirely new research fields in the 21st century. Among them, quantum metrology – the scientific study of measurement enhanced with quantum mechanics – holds the promise to advance accuracy and precision of measurements at the smallest scale.
Now, researchers at the University of British Columbia (UBC) and the British Columbia Institute of Technology (BCIT) have invented and proven a self-calibrating quantum sensor based on single atoms. “This is a very exciting result - we made a fundamental physics discovery and with it achieved a breakthrough in quantum metrology,” said Kirk Madison, principal investigator and professor at UBC Physics & Astronomy. “Because of its universal applicability to any atomic or molecular species in vacuum, we believe this represents a game changer for all scientific and industrial pursuits that require accurate vacuum measurements.” The results have been published in IOP Science, with an application to the US Patent Office.
Vacuum measurements play a key role in a range of scientific and industrial applications including residual gas analysis, semi-conductor device manufacture, and atmospheric modeling. “Atoms are exquisitely sensitive to their surroundings and constitute immutable sensor elements that never age, degrade or change with use,” said lead author and collaborator James Booth, a researcher and instructor at BCIT. “In this work we used atoms to detect other particles and thus measure the absolute pressure or particle flux in vacuum.”
The idea of using trapped atoms as a pressure or flux sensor is not new. The basic idea is that the passage of a particle nearby to a stationary sensor atom is heralded by a change of the sensor atom’s quantum state. By measuring the rate of state changes in an ensemble of sensor atoms, the flux of particles passing through the ensemble can be inferred assuming the cross-section, or characteristic range of the “perceptiveness” of the sensor atom, is known.
However, there exists a major scientific roadblock: that the cross-section, a property of the sensor atom and the interacting particle, is not known with sufficient accuracy. Calculating it accurately is a proverbial example of a hard quantum problem that is easy for a quantum computer and nearly impossible for a classical computer - except for the simplest of cases. Researchers at the U.S. National Institute of Standards and Technology (US NIST) estimate that they can perform this calculation for hydrogen hitting a lithium atom with an uncertainty of 5%.
So, the UBC-BCIT team explored a different approach. They wondered, “does the quantum nature of the sensing event somehow reveal the cross-section?”
The group discovered that the quantum state of the sensor atom after collision contains a fingerprint of the particle that hit it - specifically the momentum distribution of the sensor atom post-collision contains information about the type of interaction and the characteristic perception range. A collision or “sensing event” results in the spatial localization of the sensor atom to a region whose size is determined by the total collision cross-section. Quantum mechanics requires that this position localization be accompanied by a complementary change in the momentum distribution.
By measuring the momentum distribution of the sensor atoms, the cross-section can be found empirically. Using an ionization gauge calibrated for nitrogen gas by the US NIST orifice flow pressure standard, the UBC-BCIT group was able to prove the validity and accuracy of this new self-calibrating quantum sensor at the high accuracy of 0.5% level. This is also the first successful demonstration and validation of an atom-based pressure sensor.
“The atoms tell us everything we need to know”, said lead author Pinrui Shen, a UBC Physics & Astronomy Ph.D. student. “This work liberates the quantum pressure standard from theory!”, said co-author Roman Krems, a quantum theorist and professor of chemistry at UBC’s Stewart Blusson Quantum Matter Institute (SBQMI).
This new development positions UBC as an international leader in the field of quantum metrology for vacuum measurement. In addition, researchers at the SBQMI are planning to investigate its application to molecular bean epitaxy (MBE) - a method of thin-film deposition of crystals. “Accurate measurement and control of particle fluxes are essential for my materials research with molecular beam epitaxy, and there is no general solution to this problem that I know of,” said Ke Zou, an MBE expert and assistant professor with UBC Physics & Astronomy at SBQMI. “We are eager to test this new sensor for MBE flux control."
Media Contact
Dr. Kirk Madison
Associate Professor, UBC Physics & Astronomy
madison@physics.ubc.ca
Theresa Liao
Communications Coordinator, UBC Physics & Astronomy
communications@phas.ubc.ca; 604-822-0596
Read More
- Universality of quantum diffractive collisions and the quantum pressure standard James L Booth, Pinrui Shen, Roman V Krems, and Kirk W Madison. 2019 New J. Phys. 21 102001
- Realization of a universal quantum pressure standard Pinrui Shen, Kirk W Madison, and James L Booth. 2020 Metrologia 57 025015
- QUANTUM PRESSURE STANDARD AND METHODS FOR DETERMINING AND USING SAME MADISON; Kirk W.; (Vancouver, CA) ; BOOTH; James Lawrence; (Burnaby, CA) ; SHEN; Pinrui; (Vancouver, CA) ; KREMS; Roman V.; (Vancouver, CA). US patent publication No. 2020/0025639